One of the most common complaints when using fluorescently-labeled antibodies for staining biological samples is that the specific signal is too low. Another is that the background is too high, obscuring detection of the target. We spoke with Eric Torres, Ph.D., Marketing Manager at Biotium, Miranda Lewis, Ph.D., Marketing Manager at Jackson ImmunoResearch, and Steve Titus, Senior Manager Product Management at Thermo Fisher Scientific to learn about some of the methods being used for amplification and background reduction.
Causes of Low Specific Signal
There are many possible reasons for seeing low specific signal in a fluorescence-based immunoassay. Examples include the use of primary antibodies that are not validated for the chosen species or application, failure to fix and permeabilize samples when staining for intracellular analytes, and epitope masking due to formaldehyde fixation. In addition, photobleaching and incorrect fluorophore selection for the reader’s lasers and detectors are often to blame. However, low specific signal may also result from low analyte concentrations. In this scenario, researchers may need to consider some form of signal amplification.
Signal Amplification Methods
A broad range of signal amplification methods has been developed for antibody-based research, most of which involve increasing the number of reporter molecules at the antigen-antibody complex. The following are some of the most widely used examples:
Indirect Detection
Switching from direct detection (with labeled primary antibodies) to indirect detection (with unlabeled primary antibodies and labeled secondary antibodies) is one of the easiest ways of boosting the specific signal. Because most secondary antibodies are polyclonal, multiple secondaries can bind to a single primary antibody, as shown in Figure 1.
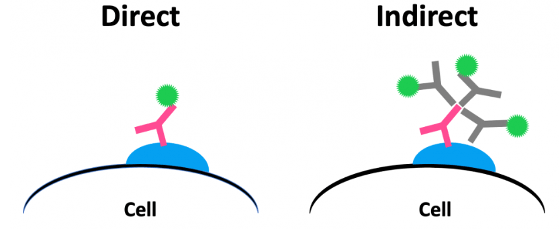
Figure 1. Direct vs. indirect detection.
Labeled-Streptavidin Biotin (LSAB) Method
The LSAB method takes indirect detection one step further with an additional reagent layer. After incubating the sample with an analyte-specific primary antibody and a biotinylated secondary antibody, a streptavidin conjugate is added, as shown in Figure 2. While LSAB was originally developed for enzyme-based detection (e.g., with HRP-streptavidin), an extensive selection of streptavidin-fluorophore conjugates is now available to researchers, including products from Biotium, Jackson ImmunoResearch, and Thermo Fisher Scientific.
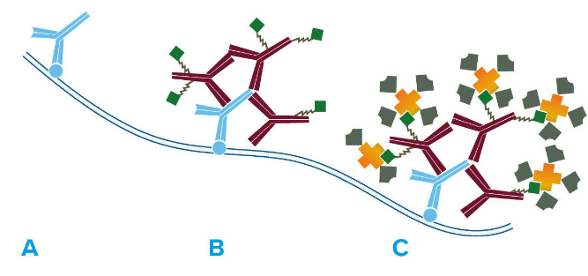
Figure 2. The LSAB method. A) Primary antibody detects target antigen. B) Biotinylated secondary antibody recognizes primary antibody. C) Streptavidin conjugate binds biotinylated secondary antibody. Image provided by Jackson ImmunoResearch.
Tyramide Signal Amplification (TSA)
TSA is based on HRP-catalyzed deposition of tyramide on and near the target of interest, as shown in Figure 3. First, a cell or tissue sample is labeled with an analyte-specific primary antibody, followed by an HRP-conjugated secondary antibody. Next, a fluorescently-labeled tyramide substrate is added. In the presence of low concentrations of H2O2, HRP converts the substrate to a highly reactive form, resulting in high density tyramide labeling. Importantly, because the fluorescent label becomes covalently bound to the sample, multicolor staining can be achieved by stripping the antibodies away and repeating the staining process with a different tyramide substrate.
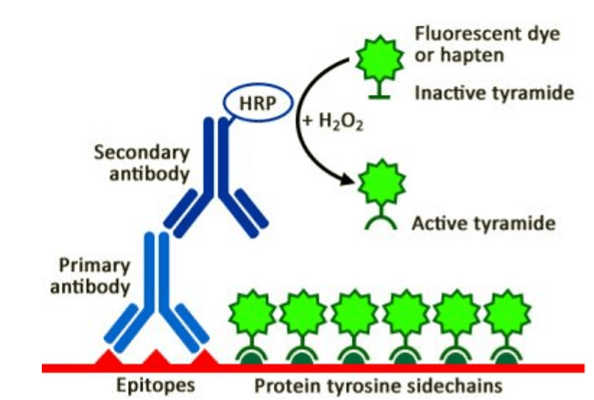
Figure 3. Tyramide signal amplification. The sample is labeled with an analyte-specific primary antibody and an HRP-conjugated secondary antibody, followed by the addition of a fluorescently-labeled tyramide substrate. HRP-catalyzed deposition of tyramide delivers high assay sensitivity. Image provided by Biotium.
Both Biotium and Thermo Fisher Scientific offer a broad array of TSA products. Biotium’s catalog includes more than 20 standalone Tyramide Conjugates, which are based on its popular CF® Dyes, as well as a range of TSA Kits containing all of the critical reagents for tyramide labeling. Recently, Biotium augmented its selection of near-IR CF® Dye Tyramides with CF®710, CF®725, and CF®740. These new CF® Dye Tyramides were developed to offer exceptional photostability, as well as address the poor chemical stability of cyanine-based near-IR dyes in oxidizing amplification buffer.
Thermo Fisher Scientific recently expanded its portfolio of TSA and other enzyme-mediated signal amplification products with Aluora™ Spatial Amplification Individual Dyes and Aluora™ Spatial Amplification Kits, which enhance sensitivity by as much as 200-fold compared with standard IHC. Cyclic staining using Aluora™ Spatial Amplification Kits is shown in Figure 4.
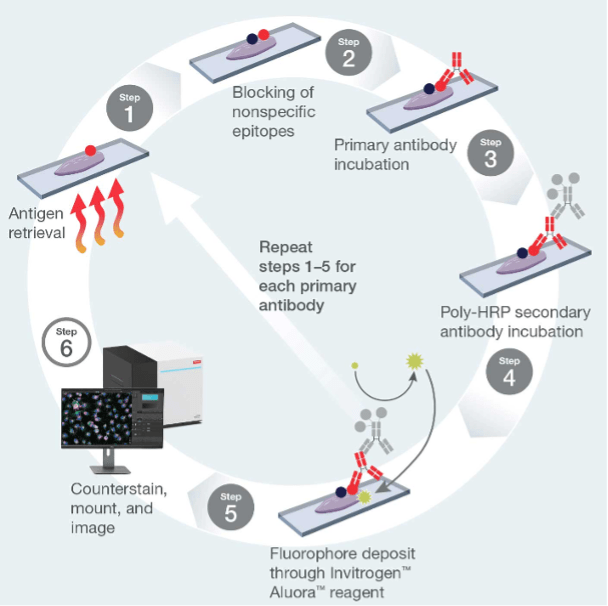
Figure 4. Cyclic staining using Aluora™ Spatial Amplification Kits.
Causes of High Background
High background can result from inadequate blocking, insufficient washing, and using too much antibody. It can also be due to autofluorescence, non-specific antibody binding, and incorrect instrument setup and imaging parameters. While assay optimization goes some way toward addressing these issues, additional background reduction techniques may be required.
Background Reduction Techniques
Background reduction techniques take many forms, depending on the assay in question. The following are some common approaches:
Fc Receptor Blocking
Incubating samples with a protein-based blocking agent, such as bovine serum albumin (BSA) or casein, serves to prevent antibody binding to non-specific epitopes. However, it may not always be sufficient to stop Fc-mediated antibody binding to the Fc receptors (FcR) found on many types of immune cells. Methods for FcR blocking include incubating samples with normal serum (i.e., serum from a non-immunized animal) or Fab fragment antibodies from the same host species as the secondary antibody. To learn how Fab fragment blocking makes species-on-species staining possible, take a look at this video from Jackson ImmunoResearch.
Autofluorescence Quenching
Autofluorescence can originate from endogenous sample components, such as collagen and lipofuscin; aldehyde fixatives like formaldehyde and glutaraldehyde; and media additives, including riboflavin and phenol red. An easy way of addressing autofluorescence is to switch fluorophores. For example, if you are detecting GFP and have autofluorescence in the green channel, you could consider using an anti-GFP antibody labeled with Alexa Fluor® 647 to avoid it.
Alternatively, you may want to think about using an autofluorescence quenching reagent. For example, lipofuscin autofluorescence can be minimized using Biotium’s TrueBlack® Lipofuscin Autofluorescence Quencher, which resolves traditional Sudan Black B’s far-red background, or its EverBrite TrueBlack® Hardset Mounting Medium, which allows for quenching lipofuscin and mounting samples in a single step. In addition, TrueBlack® Plus Lipofuscin Autofluorescence Quencher is a refinement of the original TrueBlack® product that reduces far-red background even further and can be used in aqueous media for deeper tissue penetration.
For quenching autofluorescence from non-lipofuscin sources, Thermo Fisher Scientific offers the ReadyProbes™ Tissue Autofluorescence Quenching Kit.
Use of Cross-Absorbed Secondary Antibodies
Cross-adsorbed secondary antibodies are affinity purified to remove components that bind to off-target species of immunoglobulin. They can be especially useful for multiplexed experiments, where there is potential for cross-reactivity with other antibody reagents. Additionally, when performing IHC, using a secondary antibody that is cross-adsorbed against the host species of the sample will reduce the chance of cross-reactivity with endogenous immunoglobulins. Available options include Highly Cross-Adsorbed Secondary Antibodies from Biotium, Cross-Adsorbed (min X) Secondary Antibodies from Jackson ImmunoResearch, and Superclonal Recombinant Secondary Antibodies from Thermo Fisher Scientific.
Supporting Your Research
Whichever fluorescence-based technique you are performing, FluoroFinder can help with reagent selection. Our Antibody Search function lets you quickly identify antibodies that are validated for your chosen application, while our Spectra Viewer enables you to visualize the excitation and emission spectra of different fluorophores and identify those that are distinguishable from your background.